Domestication et élevage en masse de la mouche méditerranéenne des fruits, Ceratitis capitata (Diptera: Tephritidae) de la forêt d'argane
Résumé
La mouche méditerranéenne des fruits, Ceratitis capitata (Wiedemann) (Diptera: Tephritidae), est l’un des principaux ravageurs des cultures fruitières au Maroc et dans le monde. Un élevage de masse réussi et optimisé de ce ravageur est un élément clé de plusieurs stratégies de lutte intégrée. Dans ce contexte, des colonies de mouches méditerranéennes méditerranéennes, C. capitata, issues de pupes de fruits d'argan infestées et récoltées sur le terrain ont été établies, entretenues et étudiées au laboratoire pendant 21 générations successives. Le cycle vital et les paramètres démographiques ont été suivis sur 14 générations. Par la suite, les 20e et 21e générations ont été étudiées pour évaluer respectivement la production optimale de pupes et d'oeufs. Par conséquent, différents volumes d'oeufs variant de 0,25 à 3 ml ont été ensemencés avec un régime alimentaire larvaire de 1 kg et les cages adultes ont été peuplées avec des densités de pupes de 2000 à 20 000. Notre étude a mis en évidence le potentiel d'adaptation de la mouche méditerranéenne aux conditions de laboratoire. En fait, à partir de la génération 7, tous les œufs sont pondus à travers les cages en filet au lieu du dispositif de ponte. La production d'œufs, la production de pupes, le taux d'éclosion des œufs, le taux d'émergence des pupes et la période de développement larvaire ont été améliorés au fil des générations. Nos données recommandent l’utilisation de 1,25 à 1,5 ml d’œufs par kilogramme de régime et de 16 000 chrysalides par cage afin d’optimiser la production de pupes et d’œufs. D'autres expériences, notamment en ce qui concerne la condition physique, la compétitivité de l'accouplement et la variation génétique, sont nécessaires pour étudier la stabilité des populations après des phases de captivité supplémentaires en laboratoire.
Mots clés: mouche méditerranéenne des fruits, Ceratitis capitata, Tephritidae, Domestication, élevage en masse, souche sauvage
Téléchargements
INTRODUCTION
The Mediterranean fruit fly, Ceratitis capitata, is one of the major fruit crop pests in Morocco (Mazih, 2008). The fly originated in Africa, but today presents a world-wide geographic distribution, showing great colonizing potential (Diamantidis et al., 2009).
Efficient control of C. capitata requires, in addition to combining multiple control methods in an integrated pest management strategy, adequate information regarding the pest in relation to beneficial species, as well as the dynamics of these organisms under varying environmental conditions and factors, all within an economic framework for assessment of costs and benefits (Ditomaso et al., 2017). Mass and laboratory-rearing of insects is a key component of several integrated pest management strategies (Sorensen et al., 2012). Hence, several purposes of mass rearing are known: Production of raw material for secondary product (virus, pheromones, etc), production of hosts for entomophagous species, displacement, parasites and predators, sterile insect techniques (Boller, 1972). Also, laboratory-reared flies can be useful for insect bioconversion of waste (Van Huis, 2013) or for the study of problems concerning adaptation and colonization processes, in a more general biological approach (Souza et al., 1988). In addition, insects have a large potential for sustainably enhancing global food and feed production, and commercial insect production is a rising industry of high economic value (Jensen et al., 2017).
Mass production often focuses on increasing output and deliberate or inadvertent selection can result in traits that optimize rapid growth, shorter development, and reduced or even loss of diapause under artificial conditions (Grayson et al., 2015). Within a mass-rearing facility the most important factors for efficient production of larvae are high egg production, low mortality, and a large number of fertile adults in as little space as possible (Parry et al., 2017).
For many ecologically important traits, the performance of laboratory strains can differ from wild populations and the use of artificial diet may not reflect growth and development on natural diets in some contexts (Grayson et al., 2015). Demographic studies have been completed for several species of tephritids. Population parameters have been measured for wild strains of the Mediterranean fruit fly, C.capitata, several differences between collected flies reared for 12 generations and populations maintained for more than 12 years were observed (Rössler, 1975).
The objectives of this research were to implement a laboratory adapted strain of medfly and to optimize its mass rearing output (eggs and pupae). We therefore initiated a mass rearing of wild strain medfly, collected from infested argan fruits, to reach adapted colony able to be maintained and scaled-up in the laboratory. Life history and demographic parameters of the first generation, considered as wild, and the successive generations were recorded. Further trials carried out on 14th generation, considered as laboratory-adapted, to optimize its mass rearing outputs.
MATERIALS AND METHODS
Laboratory adapted strain implementation
Wild populations were established using medfly collected from infested argan fruit in and around the Hassan II Institute of Agronomy and Veterinary Medicine, Agadir (30°35’ N, -9°47’ E). Dropped argan fruit was collected from the ground surrounding infested trees and was placed in perforated trays, which allowed emerging larvae to crawl through the holes and pupate in a sandy container below. Pupae were sieved from the sand daily and were maintained in sealed petri-dishes (200 Pupae/petri-dish) at 27˚C±2 during 7 days. Wild adults eclosed in insect rearing cages, covered with mesh, with a density of about 16000 flies per cage. This constituted the first generation (G1) or wild type mentioned in the text.
For generations 1 and 2, because of pupae availability, three cages (replicates) were used instead of four cages for the following generations. Eggs collected indifferently from diverse cages constituted the next generation. Therefore, material from different cages was mixed and all cages constituted a single population (Zygouridis et al., 2014).
Adult fly colonies were maintained in a controlled environment room (temperature: 25±1 °C, RH: 60-65%, 12:12 light:dark cycle) and were provided a standard diet of enzymatic yeast hydrolysate and sugar (1:4) together with water ad libitum.
For the first six generations, cages (30 cm × 30 cm × 30 cm) were used. Eggs were collected either in the water trays that are located under the cages or using an oviposition device. Only eggs from oviposition device are seeded in the larval diet to produce pupae. The larval diet used were slightly modified from that developed by (Tanaka et al., 1969) and consist of: Bran (259 gr), Brewer’s yeast (65 gr), Sugar (148 gr), Sodium Benzoate (2.6 gr), HCl-0.9 % (100 ml) and water (385 ml).
These devices consisted of a plastic dome with numerous small holes through which the females laid their eggs. The plastic covers a 9 cm diameter Petri dish containing a wet filter paper. One mature argan fruit was placed inside the petri dish so that its odor stimulated egg-laying. Eggs were carefully removed with a fine brush and by flushing the underside of the dome and the filter paper with water. After the eggs had sunk in the water, they are incubated for 48h and then pipetted and seeded on 1 kg of artificial diet for larval development (27–28 °C, 55% R.H.) in trays and are allowed to develop for 5-6 days (Alonso et al., 2005). Four trays (replicates) were used for each generation. When larvae begin to crawl up the side of the tray, the pupation medium is introduced underneath the larval tray and both are covered in netting to minimize escapees. Pupation trays are refreshed daily to allow synchronization of adult eclosion. 5 days post pupation the pupae are placed in cages containing food and water until eclosion.
At the 7th generation onward, when the colony seemed established, adults were maintained in 77 cm × 72 cm × 7 cm wooden cages with food and water. Oviposition devices are removed and all eggs are collected throw cages mesh. The cages were suspended on metallic trolleys.
Life history and demographic parameters
Egg production
For generations 1 to 13, records were kept on the following: 1) Preovipositional period (d), 2) Harvest duration (d), 3) Total egg production (ml), 4) Eggs collected on oviposition device (%), 5) Total Eggs/female (estimated by assuming that 1ml of eggs is equivalent of 22 100 based on previously attained data in our laboratories), 6) Eggs eclosion % (by counting a sample of 100 eggs placed on a moistened filter paper within a 90-mm Petri dish).
Pupal production
For generations 2 to 14, records were kept on the following: 1) Larval development time (d), 2) Pupal collection duration (d), 3) Total pupal production (ml), 4) Egg to pupae recovery (%) calculated based on the initial number of eggs introduced into each container of rearing medium and assuming that 100 produce pupae measure 1,5 ml and 5) Emergence (%) based on lots of 100 puparia from each replicate.
Laboratory-adapted strain mass rearing optimization
To evaluate laboratory adapted strain optimal egg production, the 20th generation was studied. Each cage was populated with different volumes of pupae; the expected male: female ratio was 1:1. Twelve cage adult population densities were analyzed ranging from 2000 to 24,000 adults per cage. Each evaluation was repeated six times for statistical replication. Egg collections started from day 6 post adult eclosion and proceeded until the colony decline.
To evaluate optimal pupal production, the 21th generation was studied. Different volumes of eggs (0.25-3.00 ml) were seeded per 1 kg of diet as described above. Six replicates for each egg volume tested were analyzed. Following pupation, the volume (ml) of pupae was measured.
Statistical analysis
Data were subjected to one-way analysis of variance (ANOVA) and, where appropriate, means were separated by Tukey’s range tests. P-values of ≤ 0.05 were considered statistically significant. Analysis was performed using the Minitab 16 software.
RESULTS
Life history and demographic parameters
Egg production
In order to reveal the plasticity of Mediterranean fruit fly, C. capitata to be adapted to laboratory controlled environment, wild strain was kept among different generations. Figure 1 shows the total volume of eggs (ml) obtained from cages populated with 16000 of wild pupae over different generations. Significant differences in total egg production were observed among the different generations (F=76,58; dl=6; p<0,001) (Figure 1). It was clear that the total egg production increases significantly over generations. During the first three generations, the production fluctuated around 26 ml. After, it increased to slightly more than 50 ml for generations 5 to 7. The best yields were reached from generation 11 onward (more than 90 ml) with a peak value of 98±5,92 on generation 13.
Table 1 shows that the period from adults’ emergence and first egg production (Preovipositional period) did not differ over the generations (F=0,42; dl=6; p=0,857). It oscillates 5,5 to 6 days. No statistically significant differences were observed among harvest durations for all generations (F=2,68; dl=6; p=0,045). It’s comprised between 14 and 15,5 days. Regarding the eclosion percentages of the eggs, it was different among generations (F=5,28; dl=6; p<0,001). The lowest value was observed for first generation (63,67 ± 2,91). However, this percentage was higher for the following generations (more than 70%).
Our data suggest that the percentage of eggs collected on the ovipositor device dropped significantly from a given generation to the next one (F=1218,89; dl=6; p<0,001). In fact, the first generation was not adapted at all to lay eggs throw cages mesh. 100 % of eggs were collected on the oviposition device. However significant changes in oviposition option were observed in the following generation. Hence, the percentage of eggs collected on oviposition device dropped to 63,98 ± 2,05 and 43,69 ± 1,23 c for generations 3 and 5 respectively. From generation 7 onward, all eggs were produced throw cages mesh.
Another parameter that shows the adaptation of medfly to laboratory conditions is the female daily fecundity. Our data suggest that the differences among the produced Egg/female/day over different generations were highly significant (F=80,84; dl=6; p<0,000). It’s clear that females of first generations produced less eggs/female/day (about 5 for generations 1 and 3). This parameter considerably increased from generation 5 onward. The best value of 17,44 ± 0,82 was recorded for generation 13.
Pupal production
Figure 2 shows the total volume of pupae (ml) obtained from 1 ml of eggs seeded into 1 kg larval diet over different generations. Significant differences in total pupal production were observed among the different generations (F=27,45; dl=6; p<0,001) (Figure 2). The total pupal production increases over generations. In fact, 1 ml of second generation eggs produced 127,78±6,81 ml. This volume reached 158,74±1,17 ml for generation 8. From Generation 10 onward, the pupal production exceeded the volume of 160 ml. Hence a peak of 175,74±3,37 was recorded for generation 13.
In table 2, larval development time shortened with about 2 days from generation 2 to generation 14 (F=7,25; dl=6; p<0,001). It moved from 13±0,00 days to 10±75 respectively. The pupal collection time remained unchanged for all generations (F=0,14; dl=6; p=0,988). Concerning the total pupal weight, it reflects the total pupal production and followed the same trend of increasing over generations (F=27,45; dl=6; p<0,001). Pupal recovery did significantly improve as it passed from 38,55 ± 2,05 % days for generation 2 to 53,01 ± 0,61 for generation 14 (F=27,45; dl=6; p<0,001). The quality of pupae, reflected by the emergence rate, was also significantly enhanced (F=36,80; dl=6; p<0,001). The first generations had an emergence rate inferior to 80 %. From generation 8 onward this rate exceeded the limit of 80 % reached 87,25 ± 1,70 % for generation 14.
Laboratory-adapted strain mass rearing optimization
Figure 3 shows the total pupal production for different seeded eggs densities tested. Significant differences in total pupal production were observed among the different densities (F=121,70; dl=11; p<0,001). The highest pupal rearing efficacy was achieved with a volume of eggs comprised between 1,25 and 1,5 ml seed in 1 kg of larval diet. Egg volumes lower or higher than the optimum recorded produced fewer pupae. The coefficient of determination (R²) between the total pupal production and pupal eggs volume, was found to be 86% (y= -4,59x2 + 56,44x + 5,55; R2=0.8617) indicating that the curve provides a good fit to the data.
Regarding egg production optimization, figure 4 shows that the total egg production differ significantly among the different pupae densities (F= 49,65; dl=11; p<0,001). The highest egg production was achieved with 16000 pupae per cage. Pupae densities lower or higher than the optimum recorded produced fewer eggs. The coefficient of determination (R²) between the total egg production and pupal density per cage, was found to be 96% (y = -1,6587x2 + 26,063 x - 12,61; R2=0. 0,9627) indicating that the curve provides a good fit to the data.
DISCUSSION
Our study highlights the potential adaptability of the Mediterranean fruit fly to laboratory conditions. In fact, there were improvements in the parameters such as egg production, pupal production, eggs eclosion rate, pupae emergence rate and different stages development time. In addition of these parameters, the main trait showing the adaptability of the strain is its ability to lay all eggs through the cages mesh instead of oviposition device. In fact, from generation 7 onward all eggs are collected from the mesh. Hence, for an insect population to adapt to a new diet, the most important behavioral modifications require the females to oviposit on the new food (Bravo and Zucoloto, 1998). Leppla et al. (1983) reported that the Mediterranean fruit fly (medfly), C capitata required more than 5 generations to strain adaptation. Methods for insect rearing on artificial diet and the availability of laboratory strains have enabled a wide range of studies that could otherwise be infeasible (Grayson et al., 2015). The lack of success during the early stages of colonization suggests that the wild insects have to be adapted to laboratory conditions and that intense selection occurs in the F 1 and following generations. However, it was difficult to determine if all insects have contributed to the process of adaptation during the first generations (Boller, 1972). For example, Selection on strains of the gypsy moth, Lymantria dispar (L.), maintained on laboratory, has enhanced a variety of traits, resulting in faster development, shorter diapause, and greater fecundity (Grayson et al., 2015). Also, the laboratory strain of Caribbean fruit flies Anastrepha suspensa (Loew) (Diptera:Tephritidae), began mating at an earlier age and mated more rapidly is small screen cages in the laboratory conditions (Mazomenos et al., 1977)
Our results demonstrate that the preoviposition period (about 6 days) and oviposition period (about 15 days) remained unchangeable over generations. The same observation was reported bay (Diamantidis et al., 2011) for Kenyan flies. Assuming that the 11th generation onward could be considered as laboratory adapted strains, these results are different from (Foote and Carey, 1987) and (Vargas and Carey, 1989) that reported that females in the laboratory population produced eggs at a significantly younger age than those in the wild populations.
For egg production, the total egg production increases significantly over generations. This finding is confirmed by different authors. Vargas and Carey (1989) found that egg production by the laboratory population was significantly greater than by all wild populations. The laboratory-adapted strain produced about 5 times more eggs which is the same relation reported by Bravo and Zucoloto (1998).
The number of egg laid per female increased from 5,02 ± 0,39 for generation 2 to 17,44 ± 0,82 for generation 13. Neto et al. (2012) reported that in similar large-scale experiments, 18,000 VIENNA-8 TSL (Temperature Sensitive Lethal) adults per cage, yielded 7.54 ± 1.70 eggs per female per day, for a period of 10 days. Blay and Yuval (1999) reported the greatest number of eggs laid by a mated female per day to be 94 eggs.
With respect to the hatch rate of eggs collected from the mass-rearing cages, it was slightly improved over generation. During the 8 generations tested, Rempoulakis et al. (2016) found this parameter to remain stable.
There was significant improvement of quantitative and qualitative parameters such as pupal production, pupal emergence rate and larval development period during domestication process of the Mediterranean fruit fly in laboratory conditions. This is due to that fact that at least a certain percentage of immature accepted the new food and ingest it in adequate amounts (Bravo and Zucoloto, 1998). Our findings are conformed by Vargas and Carey (1989) that reported that the duration of the larval stage differed significantly between laboratory and wild lines of flies. In fact, C. capitata is known for its plasticity that permits their adaptation to new habitats, reflected in its present world-wide geographical distribution (Souza et al., 1988).
The improvement of mass rearing performances should not lead to strains completely different from the initial one. Rodriguero et al., (2002) reported that copulatory success might be affected between wild and laboratory adapted strain, as a side effect due to selection of wild males over lab males due to differential sexual activity or other causes. Indeed, Certain level of sexual incompatibility was observed between strains under both laboratory and field conditions of the Mexican fruit fly, Anastrepha ludens (Loew) (Meza-Hernandez and Díaz-Fleischer, 2006). To counter any unwanted changes, there is a need to gain knowledge of the basic biology of the organism in question, including the basic population genetics and the effects of temperature, diet, and density (Jensen et al., 2017). It is therefore of fundamental importance to follow the adaptation process genetically and try and link the strain’s mating efficiency and ‘wild’ character with genetic markers (Zygouridis et al., 2014)
CONCLUSION
In conclusion, our study shows that wild Mediterranean fruit fly, C. capitata collected from infested argan fruits were well established in laboratory. Different population traits, such as egg production, pupal production, eggs eclosion rate, pupae emergence rate and larval development period, were improvement over the fourteen studied generations. Once adapted the laboratory conditions, the optimization of the mass rearing parameters is required as it influences insect’s quality and production cost. Our findings provide important insights of the optimal insects’ densities to use for eggs and larval production. Obviously, further studies are required to characterize occurring changes over different generations.
REFERENCES
Alonso M., Del Río M.Á., Jacas J.A. (2005). Carbon dioxide diminishes cold tolerance of third instar larvae of Ceratitis capitata Wiedemann (Diptera: Tephritidae) in ‘Fortune’ mandarins: implications for citrus quarantine treatments. Postharvest biology and technology, 36: 103-111.
Blay S., Yuval B. (1999). Oviposition and fertility in the Mediterranean fruit fly (Diptera: Tephritidae): effects of male and female body size and the availability of sperm. Annals of the Entomological Society of America, 278-284.
Boller E. (1972). Behavioral aspects of mass-rearing of insects. Entomophaga, 17: 9-25.
Bravo I.S.J., Zucoloto F.S. (1998). Performance and feeding behavior of Ceratitis capitata: comparison of wild population and a laboratory population. Entomologia experimentalis et applicata, 67-72.
Diamantidis A.D., Carey J.R., Nakas C.T., Papadopoulos, N.T. (2011). Ancestral populations perform better in a novel environment: domestication of Mediterranean fruit fly populations from five global regions. Biological journal of the Linnean Society, 102: 334-345.
Diamantidis A.D., Papadopoulos N.T., Nakas C.T., Wu S., Müller H.G., Carey J.R. (2009). Life history evolution in a globally invading tephritid: patterns of survival and reproduction in medflies from six world regions. Biological journal of the Linnean Society, 97: 106-117.
Ditomaso J.M., Van Steenwyk R.A., Nowierski R.M., Vollmer J.L., Lane E., Chilton E., Burch P.L., Cowan P.E., Zimmerman K., Dionigi C.P. (2017). Enhancing the effectiveness of biological control programs of invasive species through a more comprehensive pest management approach. Pest management science, 73: 9-13.
Foote D., Carey J.R. (1987). Comparative demography of a laboratory and a wild strain of the oriental fruit fly, Dacus dorsalis. Entomologia experimentalis et applicata, 44: 263-268.
Grayson K.L., Parry D., Faske T.M., Hamilton A., Tobin P.C., Agosta S.J., Johnson D.M. (2015). Performance of wild and laboratory-reared gypsy moth (Lepidoptera: Erebidae): A comparison between foliage and artificial diet. Environmental entomology, 44: 864-873.
Jensen, K., Kristensen, T.N., Heckmann, L.-H. and Sørensen, J.G. (2017). Breeding and maintaining high-quality insects. Insects As Food and Feed. pp. 175-198. Wageningen Academic Publishers.
Leppla N., Huettel M., Chambers D., Ashley T., Miyashita D., Wong T., Harris E. (1983). Strategies for colonization and maintenance of the Mediterranean fruit fly. Entomologia experimentalis et applicata, 33: 89-96.
Mazih A. (2008). Current situation of citrus pests and the control methods in use in Morocco. International Conference on Integrated Control in Citrus Fruit Crops. (ed. F. García-Marís), pp. 10-16. IOBC/wprs, Catania, Italy.
Mazomenos B., Nation J., Coleman W., Dennis K., Esponda R. (1977). Reproduction in Caribbean fruit flies: comparisons between a laboratory strain and a wild strain. Florida Entomologist, 139-144.
Meza-Hernandez J.S., Díaz-Fleischer F. (2006). Comparison of sexual compatibility between laboratory and wild Mexican fruit flies under laboratory and field conditions. Journal of Economic Entomology, 99: 1979-1986.
Neto S., Santos T.R.D.O., Dias V.S., Joachim-Bravo I.S., Benevides L.D.J., Benevides C.M.D.J., Silva M.V.L., Dos Santos D.C.C., Virgínio J., Oliveira G.B. (2012). Mass-rearing of Mediterranean fruit fly using low-cost yeast products produced in Brazil. Scientia Agricola, 69: 364-369.
Parry, N.J., Pieterse, E. and Weldon, C.W. (2017). Longevity, fertility and fecundity of adult blow flies (Diptera: Calliphoridae) held at varying densities: Implications for use in bioconversion of waste. Journal of economic entomology, 110: 2388-2396.
Rempoulakis P., Taret G., Ul Haq I., Wornayporn V., Ahmad S., Tomas U.S., Dammalage T., Gembinsky K., Franz G., Cáceres C. (2016). Evaluation of Quality Production Parameters and Mating Behavior of Novel Genetic Sexing Strains of the Mediterranean Fruit Fly Ceratitis capitata (Wiedemann)(Diptera: Tephritidae). PLOS ONE, 11, e0157679.
Rodriguero M., Vilardi J., Vera M., Cayol J., Rial E. (2002). Morphometric traits and sexual selection in medfly (Diptera: Tephritidae) under field cage conditions. Florida Entomologist, 85: 143-149.
Rössler Y. (1975). Reproductive differences between laboratory-reared and field-collected populations of the Mediterranean fruit fly, Ceratitis capitata. Annals of the Entomological Society of America, 68: 987-991.
Sorensen J.G., Addison M.F., Terblanche J.S. (2012). Mass-rearing of insects for pest management: challenges, synergies and advances from evolutionary physiology. Crop Protection, 38: 87-94.
Souza H.M.L., Matioli S.R., Souza W.N. (1988). The adaptation process of Ceratitis capitata to the laboratory analysis of life‐history traits. Entomologia experimentalis et applicata, 49: 195-201.
Tanaka, N., Steiner, L., Ohinata, K. and Okamoto, R. (1969). Low-cost larval rearing medium for mass production of oriental and Mediterranean fruit flies. Journal of Economic Entomology, 62: 967-968.
Van Huis A. (2013). Potential of insects as food and feed in assuring food security. Annual Review of Entomology, 58: 563-583.
Vargas R.I., Carey J.R. (1989). Comparison of demographic parameters for wild and laboratory-adapted Mediterranean fruit fly (Diptera: Tephritidae). Annals of the Entomological Society of America, 82: 55-59.
Zygouridis N., Argov Y., Nemny‐Lavy E., Augustinos A., Nestel D., Mathiopoulos K. (2014). Genetic changes during laboratory domestication of an olive fly SIT strain. Journal of applied entomology, 138: 423-432.
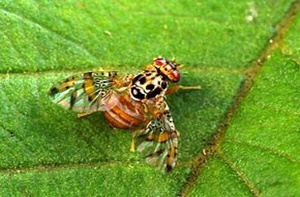
Téléchargements
Publié-e
Comment citer
Numéro
Rubrique
Licence
Revue Marocaine des Sciences Agronomiques et Vétérinaires est mis à disposition selon les termes de la licence Creative Commons Attribution - Pas d’Utilisation Commerciale - Partage dans les Mêmes Conditions 4.0 International.
Fondé(e) sur une œuvre à www.agrimaroc.org.
Les autorisations au-delà du champ de cette licence peuvent être obtenues à www.agrimaroc.org.