Antagonisme potentiel de certaines souches de Trichoderma isolées du sol marocain contre trois champignons phytopathogènes de grande importance économique
Résumé
Dans cette étude, 17 souches de Trichoderma on été isolées à partir d’échantillons du sol de différentes origines (champs et forêt d’arganier). Les espèces ont été identifiées par la caractérisation moléculaire des cultures monospores des isolats de Trichoderma et leur potentiel d’antagonisme a été évalué contre trois champignons phyto-pathogènes telluriques (Fusarium oxyxporum, verticillium dahlia et rhizoctonia solani). Après l’extraction de l’ADN, le facteur d’élongation et de traduction tef1 a été utilisé comme marqueur moléculaire pour amplifier, séquencer et par la suite caractériser les 17 souches qui ont été comparées avec leur ex-types déjà référencés. Par conséquent, l’identification moléculaire par tef1 a permis la caractérisation de trois espèces de Trichoderma à savoir T. afroharzianum, et T. guizhouense appartenant à la branche Harzianum, et T. longibrachiatum appartenant à la branche Longibrachiatum. Les 17 isolats candidats ont été sélectionnés par une méthode de criblage basée sur la PCR (polymerase chain reaction). L’évaluation du potentiel antagoniste des souches de Trichoderma contre les champignons phytopathogènes telluriques (F. oxysporum, R. solani et V. dahliae) a été réalisée par la méthode de confrontation sur boîtes de pétrie. L’étude in vitro de l’activité antagoniste par le «Pourcentage of Radial Inhibition Growth» (PRIG %) a permis de suivre l’évolution de la croissance radiale du mycélium des différents champignons phytopathogènes. Le pourcentage le plus élevé (PRIG% = 98%) a été observé pour la confrontation entre l’isolat 8A2.3 et R. solani et le pourcentage le plus faible (PRIG% = 67%) a été observé pour la confrontation entre l’isolat T9i10 et F. oxysporum. D’un autre coté, T9i12 qui est un isolat de l’espèce T. reeseia a engendré une inhibition élevée de la croissance radiale du mycélium des différents pathogènes.
Mots clés: Trichoderma spp, Fusarium oxysporum, Verticillium dahlia, Rhizoctonia solani, champignons antagonistes.
Téléchargements
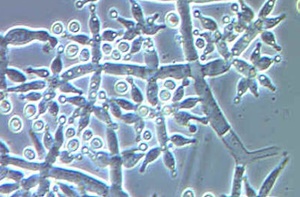
Publié-e
Comment citer
Numéro
Rubrique
Licence
Revue Marocaine des Sciences Agronomiques et Vétérinaires est mis à disposition selon les termes de la licence Creative Commons Attribution - Pas d’Utilisation Commerciale - Partage dans les Mêmes Conditions 4.0 International.
Fondé(e) sur une œuvre à www.agrimaroc.org.
Les autorisations au-delà du champ de cette licence peuvent être obtenues à www.agrimaroc.org.